QUESTIONS FOR ENERGY DEMOCRACY: Where do we get energy from, and how? What are the social and environmental implications of this?
This section discusses:
- Primary energy
- The meaning of renewable and sustainable energy
- Technical considerations for energy generation
Technologies for moving, storing and using energy are discussed in their own sections.
Primary energy
All of our energy comes in initially from a few forms of primary energy (see physics of energy):
- Nuclear reactions
- nuclear power
- the sun – a huge nuclear reactor
- solar photovoltaic electricity generation
- concentrated solar power using heat from the sun to make electricity
- direct use of heat from the sun
- biomass – plants which store sunlight through photosynthesis, other biological matter made from eating plants
- fossil fuels – buried biomass from ancient photosynthesis
- wind power – atmospheric currents are powered by solar heating
- wave power – mostly caused by the wind acting on the ocean
- hydroelectric power – the sun vaporises water and it falls as rain drained by rivers – this is the water cycle
- Gravity
- tidal power due to the interaction of the moon’s gravity with the oceans
- underwater sea currents – caused partly by solar heating, and partly by tides and the spin of the earth.
As you can see, the vast majority of our energy comes from the sun
Technologies do not ‘create’ energy. They convert it from one form to another. Some of the forms of energy we use can be stored in solid/liquid/gas form in tanks or heaps or stacks (e.g. fossil fuels, nuclear fuel, biomass). Other forms of energy are currently flowing or moving, such as the wind and the waves. Our technologies release, capture and transform energy and turn it into forms that we can use to satisfy our needs.
The UK energy flow chart (or ‘sankey diagram’) was introduced in the section on the physics of energy. It is a good way to visualise the primary energy going into the system on an annual basis. The 2017 energy flow chart below shows that a large part of our energy came from petroleum, followed by natural gas. The ‘electricity’ input is not strictly primary energy – this is made up of nuclear (the bigger light pink part) and hydro, wind, solar, and imports.
The graphs below show the primary energy sources used to produce electricity in 2016. The transmission connected capacity shows the maximum amount of energy that could be produced at one moment (power), and the generation output shows the total amount of primary energy used in 2016 (energy).
Note that ‘pump storage’ does not feature in the generation output graph because it stores electricity that originally was generated from another form of primary energy. Coal is 19.9% of capacity, but only 10% of generation output, but not in the transmission connected capacity – this is because the generators are in other countries.
Technologies for converting primary energy
If you want to understand the details of how different energy technologies work, there are a lot of resources available on the internet and in textbooks, and many courses available online and in person, from DIY solar power or wind turbine making to engineering degrees. The No Nonsense guide to Renewable Energy by Danny Chivers is a good starting point for understanding renewable energy.
This guide does not aim to repeat that information. The focus here is on how different forms of energy generation interact with the energy system, with a bit on their social and environmental impacts.
Renewable and sustainable energy
Primary energy can be ‘renewable’ or ‘non-renewable’. Renewable resources will be replenished after we have used them – for example, more solar energy will reach the earth tomorrow, however much we use today. Non-renewable resources are not replenished – when coal is gone, it’s gone.
For some renewables it is important to think about the timescales of renewal. Biomass, for example, is renewable over a meaningful human timescale – we can grow more trees or other plants to replace those we burn. However, if we use them up faster than they can grow, this isn’t sustainable.
Sustainable energy is a complex concept, which can include questions of ethics, social impact, and multiple types of environmental impact (e.g. on water quality, biodiversity, air pollution).
Sustainability considerations for all forms of primary energy include:
- land use per MWh,
- compatibility with other simultaneous land uses,
- international social and environmental impact, including for example the global impacts of coal mining
- greenhouse gas emissions
- impact of construction – whole life impact assessment
Fossil fuels are a form of ‘buried sunshine’, and burning them releases carbon dioxide into the atmosphere that was stored under the ground as an essential part of the high-oxygen atmosphere in which human life has flourished. This is not compatible with a sustainable future. Nuclear power is seen by by some as a source of low or zero carbon energy, but there are serious concerns about dealing with the waste in the long term. This guide envisions a future where renewable energy plays a major role.
Technical considerations for electricity generation
The electricity system needs to balance the electricity going into and out of the network at every moment. This is discussed in detail in the section on energy storage and transportation infrastructure. Different types of generation have characteristics which make this balancing more or less easy.
The sources of electricity generation have changed over the years:
Variability, Flexibility and predictability
Important characteristics of electricity generators, that affect balancing, include:
- Controllability: whether they can be controlled by humans
- Flexibility: how quickly and easily they can be ramped up and down to respond to varying demands.
- Variability: how much they change based on weather or other factors
- Predictability: how sure we can be of their output in advance e.g. through weather forecasts
Controllable generation is sometimes called ‘firm’ or ‘dispatchable’. Both ‘firmness’ and ‘dispatchability’ are slightly unsatisfactory terms, as dispatchability implies a high level of flexibility, and firmness implies a very steady and reliable form of generation.
The table below shows the energy sources that are controllable or variable, and indicates their predictability (if variable) and flexibility (if controllable). This is an indicative table. For some of these energy resources, the detail of how they are designed and operated makes a difference.
Resource | Controllable or Variable? | Predictability | Flexibility |
Biomass | Controllable | N/A | Medium |
Geothermal | Controllable | N/A | Depends on operation |
Hydroelectric dam | Controllable | N/A | High |
Hydroelectric run of river | Variable | Medium | N/A |
Solar power | Variable | Medium | N/A |
Tidal power | Depends on operation | Very high | Depends on design |
Wave power | Variable | Medium | N/A |
Wind power | Variable | Low | N/A |
Nuclear | Controllable | N/A | Low |
Coal | Controllable | N/A | Medium |
Gas | Controllable | N/A | High |
Wind power is highly variable but needs advanced weather forecasts to be predictable. Solar power is even more variable (there is none at all at night), but follows quite predictable seasonal and daily patterns, although it is also affected by clouds moving across the sky. Tidal power also varies a lot, but is totally predictable, as tide timetables can be calculated years in advance. Tidal power stations with lagoons can be designed to allow a high level of discretion in when they generate. Biomass can be stored and burned at will, and is thus dispatchable, but most biomass boilers or power stations are relatively slow to ramp up. Nuclear power generation levels are controlled by humans, but need to be on at a steady rate rather than being responsive to changing demands. Hydropower can be released quickly, and so is one of the most flexible sources of generation.
Patterns of variability
Wind power and solar power have variability that is affected by the weather, and can be predicted by meteorological forecasting – i.e. weather forecasts. Wind and solar generation profiles for the UK in 2015 are shown in Figure 6.
Figure 6: wind and solar PV electricity generation profiles, from 2015 data
Other important technical considerations for electrical generation
As described in the ‘physics of electricity‘ chapter, electricity is usually generated by spinning a mass inside a magnet. The mass is made to spin by:
- Energy from wind, waves or tide
- Burning fuel (usually but not always a fossil fuel) to create hot air or gas that drives a turbine – this is called ‘thermal generation’.
Traditionally, electricity has mostly been generated in big thermal power stations, burning coal, gas or another fuel to produce steam or high pressure gas which turns very large turbines. Renewable energy has different characteristics, which can be challenging for the system.
Big power stations provide stability
In an AC system, the speed of rotation of the mass is directly related to the frequency of the electricity. This means that when several spinning-mass based generators are connected, frequency must be kept the same throughout the system. At the same time, the inertia of the spinning masses themselves can help to stabilise the grid frequency. Big thermal generators are particularly well-suited to providing this stability, as they are heavy. As we move away from fossil fuels and towards renewable energy generation, other forms of inertia may be needed in the system. Wind turbines do not necessarily provide useful stability to the system, as the spin speed varies with wind speed, and they are lighter than big thermal power stations. Modern big wind turbines are now being designed with electronic controls that enable them to provide grid stability via “synthetic inertia“.
Thermal power stations ALWAYS produce waste heat
In thermal power stations, the conversion of chemical energy in the fuel to mechanical energy spinning the turbine involves significant loss of heat. There is no way around this, because of the fundamental physics of thermodynamics.
Turning heat from combustion into mechanical energy of a turbine involves a ‘thermodynamic cycle‘, and is driven by a temperature difference between the start and end of the cycle. That’s why power stations either have big cooling towers or are sited next to a body of cold water, to maintain that temperature difference.
It is not possible to improve efficiency of a thermodynamic cycle beyond the maximum thermal efficiency.
Modern ‘combined cycle gas turbines’ use the discarded heat from the first stage of power generation to power a second stage, and so reach much higher energy efficiencies. However, there is still always waste heat. This can achieve an overall energy efficiency across the two cycles of up to 60%. On the other hand, an open cycle gas turbine or coal power station typically has an efficiency of under 40%, and discard 60% of the energy in the fuel as heat.
One approach to reducing this wastage is to pipe the hot water or steam into a district heating system to heat homes or for industrial processes. Another is to switch from thermal generation to direct mechanical generation through wind, hydro or wave power. Avoiding these losses altogether is not an option.
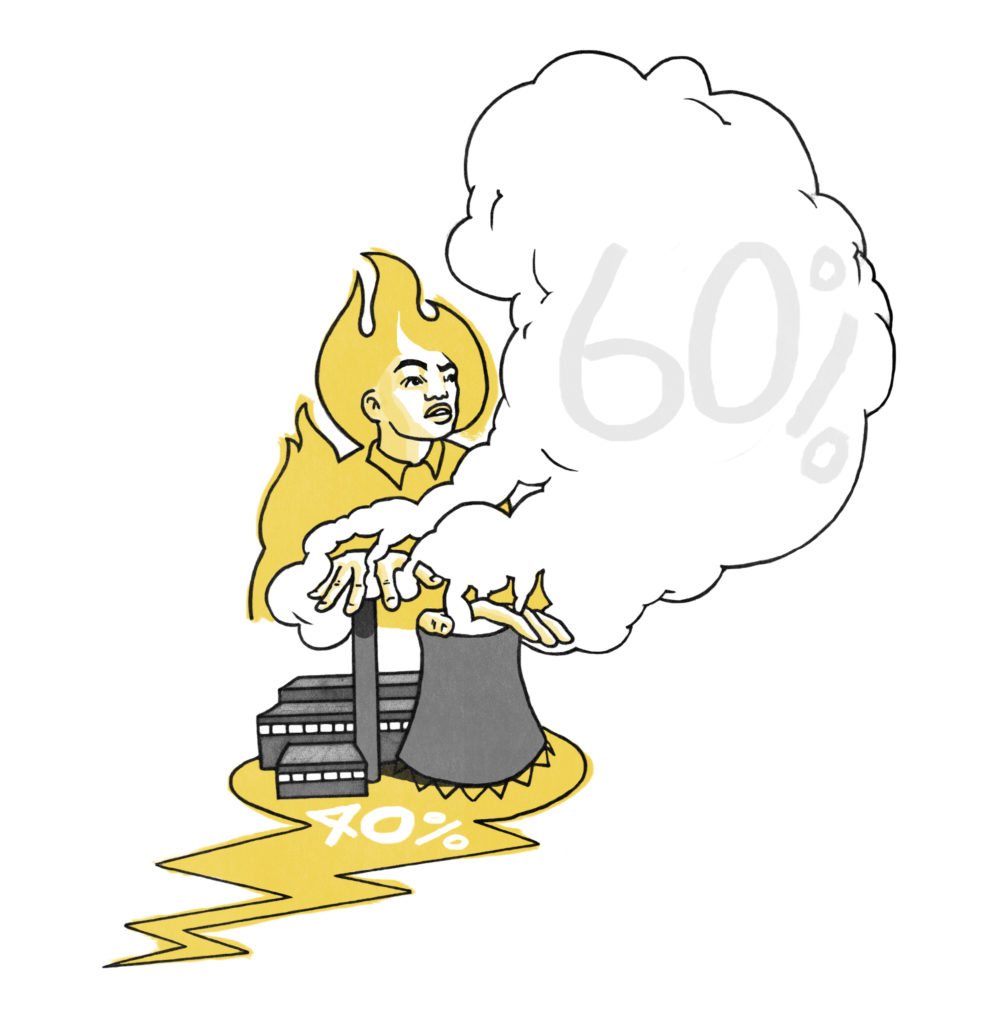
This issue also applies to internal combustion engines used in vehicles, which is one of the reasons that electric cars can achieve big reductions in energy consumption (depending on where the electricity comes from).
The science of energy conversion and losses is described in the physics of energy section.
Every energy conversion has a loss
Waste heat is an example of a more general condition. None of the processes for power generation convert mechanical energy to electrical energy with perfect efficiency. Some energy will always be lost, as we know from the second law of thermodynamics.
We should thus avoid converting from one form of energy to another where possible. Using the energy in flowing water to directly move machinery, rather than to move machinery that generates electricity which is then used to move machinery would be more efficient. Or directly using water power to compress air, and using compressed air based power tools rather than electrical power tools. This kind of thinking can lead to ‘elegant solutions’ which engineers find beautiful. On the other hand, electricity has the virtue of being versatile and easy to transport across long distances if you have a national grid (which we do in GB).
Traditional water mills that use the energy of a running stream to grind grain or make oil are elegant in this way. Some are still in use and have been updated, like this water powered walnut oil mill in the south of France.
Shaft connected to water wheel and millstone grinding walnut oil
LESSONS FOR ENERGY DEMOCRACY:
- continued use of fossil fuels is not compatible with climate stability
- every energy conversion has a loss
- thermal power stations provide stability to the electricity system, but always produce waste heat.
- shifting to renewable energy creates challenges for the energy system, particularly in terms of ‘dispatchable’ electricity and matching time of use and generation of electricity